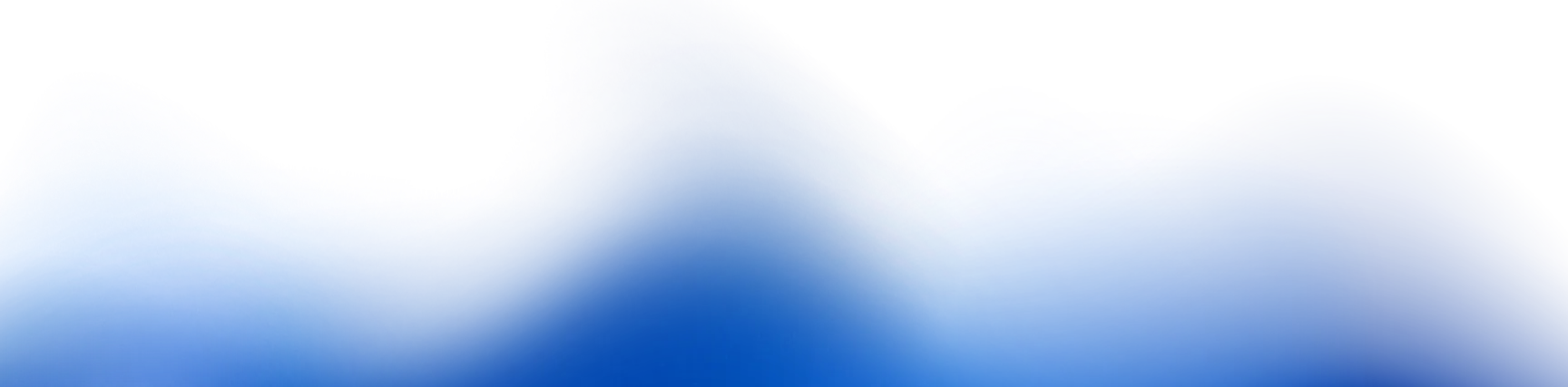
Paradromics CEO Matt Angle speaks with Stuart Cogan, Vanessa Tolosa, Thomas Stieglitz, and Loren Rieth about how to protect neural implants from the harsh environment of the body. This discussion is all about longevity and endurance, and, fittingly, it’s almost 2 hours long. Loren leaves early for a faculty meeting–wonder if his colleagues know that he came straight from the pub?
03:27 | UTD Neural Interfaces Lab
03:39 | EIC Labs
03:59 | Cogan’s highly-cited review paper
04:16 | Lawrence Livermore National Lab
04:56 | Rieth Lab at the Feinstein Institute
05:18 | Loren’s work with the Utah Array
05:39 | Human peripheral nerve stimulation
05:58 | Preclinical Vegus Nerve stimulation
06:11 | Stieglitz Lab
06:22 | Flexible Electrodes
06:41 | Long Lasting Electrodes
07:41 | Jerry Loeb: Materials Legend
08:29 | Phil Troyk
09:24 | North American Neuromodulation Society
10:44 | Melosh Lab at Stanford
12:53 | Packaging Development
17:02 | Helium Leak Test
19:01 | Work by Pancrazio
21:34 | Finetech-Brindley Stimulator
29:05 | Emerging technology @ University of Sydney
33:10 | Calvin and Hobbes
34:12 | Revolutionizing Prosthetics
35:00 | Canned Utah Array
35:35 | Flip-chip connecting
36:04 | Nick Donaldson: Mr. Clean
36:47 | Failure mode analysis
36:55 | Scaling up the Utah Array
37:54 | DARPA’s NESD Program
38:28 | High density Utah Array
39:52 | The Michigan Probe
40:00 | Vanessa’s work with Loren Frank
42:05 | Parylene C encapsulation
42:56 | Thin film
44:15 | Clean rooms
46:50 | NeuroRoots
47:28| Test structures
49:17| Implant size
50:35 | Testing strategies
52:40 | NeuroNexus
53:59| Tissue response studies
54:27 | Cogan Lab’s work on Silicon Carbide
56:10 | DARPA’s HAPTIX Program
56:30 | Reactive Accelerated Aging (RAA)
58:15 | RAA with hydrogen peroxide
58:55 | Deep Brain Stimulation
01:02:55 | Hydrolysis
01:09:00 | Silicon Carbide device
01:10:26 | Neuropixels collaboration
01:19:05 | Atomic Layer Deposition
01:26:55 | Focused research orgs
01:36:14 | Second Sight
01:43:48 | Search for Paradise by Jens Naumaunn