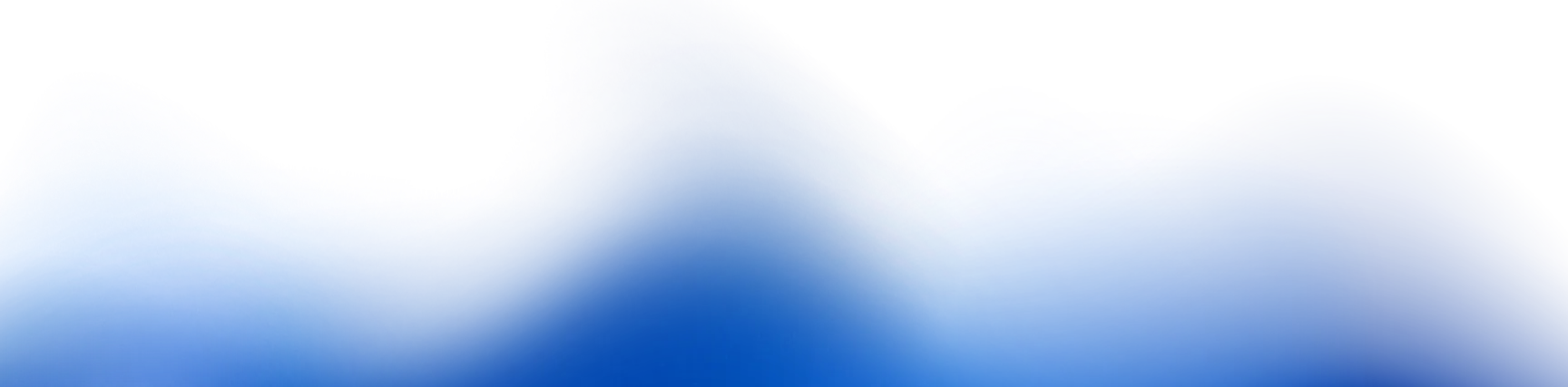
We’re back with Part II of our two-part series on Connectomics!
In part one we speculated on the legal and ethical implications of emerging technologies in the connectomics field. In part two, we don our lab coats and take a deep dive into the latest research tools, from fixation protocols for the preservation of neural tissue, to multimodal imaging techniques, to the machine intelligence designed to interpret massive data sets and reconstruct the vast neural circuits that make up the connectome.
Our guests are:
- Kenneth Hayworth, PhD, President and Co-Founder of the Brain Preservation Foundation, Senior Scientist at the Howard Hughes Medical Institute’s Janelia Farm Research Campus (JFRC)
- Robert McIntyre, CEO at Nectome
- Jeremy Maitin-Shepard, Software Engineer–Connectomics at Google
In this episode, Ken and Robert from part one return to the pub, and we are also joined by Jeremy Maitin-Shepard, an engineer and researcher at Google, who shares insights into some of the machine intelligence modalities being used to decode previously uncharted neural networks. Check out Jeremy’s recent paper on BioRxiv, as well as his published work at Google.
If you missed part one, you can listen and explore the show notes here. Cheers!
Show Notes:
0:00 | Intro
1:03 | Kenneth Hayworth, PhD
1:12 | Robert McKintyre, CEO, Nectome
1:17 | Jeremy Maitin-Shepard, PhD
1:51 | Setting the record straight
3:09 | The nucleotide sequence of bacteriophage φX174
4:22 | Frozen Zoo at San Diego Zoo
12:01 | Glutaraldehyde and reduction techniques for immunolabeling
17:39 | SWITCH Framework
19:14 | Population Responses in V1 Encode Different Figures by Response Amplitude
Permeabilization-free en bloc immunohistochemistry for correlative microscopy
19:57 | Synaptic Signaling in Learning and Memory
Structure and function of a neocortical synapse
Engineering a memory with LTD and LTP
Synapse-specific representation of the identity of overlapping memory engrams
20:28 | Ultrastructure of Dendritic Spines
Structure–stability–function relationships of dendritic spines
24:25 | Reconstructing the connectome
24:32 | Connectomics Research Team at Google
24:55 | Google x HHMI: Releasing the Drosophila Hemibrain Connectome
28:38 | Serial Block-Face Scanning Electron Microscopy
29:22 | Automated Serial Sections to Tape
29:45 | Mapping connections in mouse neocortex
30:59 | A connectome and analysis of the adult Drosophila central brain
32:14 | Expansion Microscopy
34:37 | The future of connectomics
49:49 | Mice and rats achieve similar levels of performance in an adaptive decision-making task
Want More?
Follow Neurotech Pub on Twitter